An Everyday DNA blog article
Written by: Sarah Sharman, PhD
Illustrated by: Cathleen Shaw
We express ourselves in many different ways– from the clothes we wear to the facial expressions we make. For me, I like to bring my fun, creative side across with my accessories. I really love finding colorful, fun jewelry that can help brighten up my outfits and show my personality.
It turns out our cells also express themselves. However, I don’t mean that your cells put on tiny top hats when they’re trying to be fancy. Every cell in your body contains your entire DNA code, yet different cells look different and perform different tasks. How do they do this if they all contain the same code? The answer is gene expression.
What is gene expression?
DNA is the genetic blueprint of most organisms on Earth. It contains the instructions for living things to develop, grow, and function. Gene expression is the process by which the information encoded in small units of DNA (genes) is turned into a functional product, most commonly a protein. For example, my hair is brown because genes provide instructions to produce a protein called melanin, which is involved in hair pigmentation.
So, how does DNA make these proteins? During a process called transcription, the DNA sequence of the gene is copied to make an RNA molecule. The DNA is rewritten from DNA code letters (A, C, T, and G) into a similar RNA ‘alphabet’ (A, C, U, and G) by an enzyme called RNA polymerase. In order to transcribe the DNA to messenger RNA (the specific RNA molecule in eukaryotes like humans), RNA polymerase binds to specific sections of DNA near the gene called promoters. RNA polymerase can only attach to the promoter with the help of proteins called transcription factors.
After DNA is transcribed into mRNA, the mRNA is “decoded” to build a protein. Each 3-letter combination of mRNA bases specifies one of twenty amino acids that are the building blocks of proteins. Each protein consists of many amino acids. There are more than 20,000 proteins that work together to perform all of our bodies’ functions. Not all genes provide instructions for proteins, though. Some provide instructions to build functional RNA molecules that play roles in translation, regulatory RNAs, and non-protein coding RNAs that change the expression of other genes.
When a gene is “on” and its protein or RNA product is being made, scientists say that gene is being expressed. Not all the genes in your body are turned on at the same time or in the same cells or parts of the body. The thousands of genes expressed in a particular cell determine what that cell can do. Gene expression can be thought of as a dimmer light switch, controlling when and where RNA molecules and proteins are made, as well as how much of those products are made.
How is gene expression regulated? 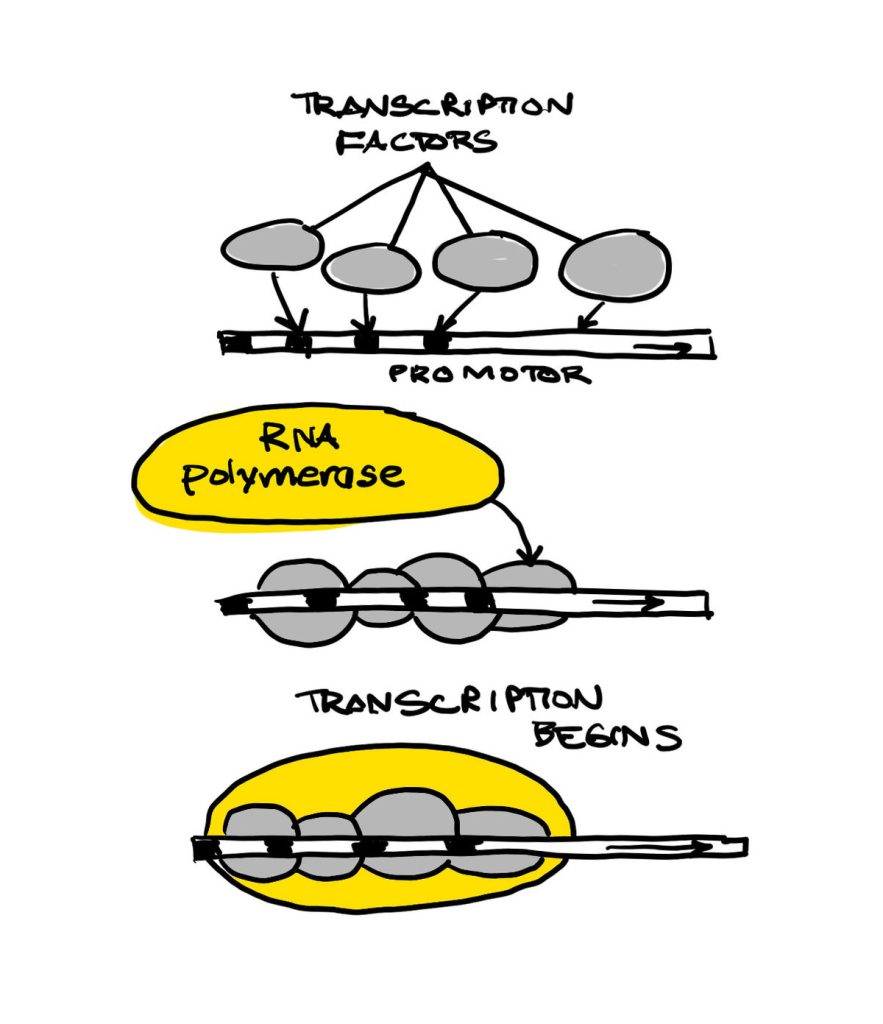
Almost all of the cells in your body contain the exact same DNA, yet you have thousands of different cell types that perform different functions. Gene regulation is how a cell controls which genes are expressed out of the many genes in its genome. The precise regulation of gene expression allows cells to adopt and maintain their specialized roles. For example, a liver cell expresses genes related to liver function, while a muscle cell expresses genes related to muscle contraction.
Gene expression can be regulated at many steps during the journey from DNA to protein. Different genes are regulated at different points, and it’s not uncommon for important or powerful genes to be regulated at multiple steps. The main control point for many genes is transcription, which we’ll focus on for the remainder of this article.
Transcription factors are proteins that help turn specific genes “on” or “off” by binding to nearby DNA. They act like a control panel for gene expression. Some transcription factors are activators, boosting a gene’s transcription, while others are repressors, decreasing transcription. They help ensure that the right genes are expressed in the right cells of the body at the right time and in the right amounts to meet the needs of the cell or organism.
Some transcription factors are generalized, serving to help RNA polymerase bind to DNA. However, large classes of transcription factors control the expression of specific, individual genes. Transcription factors bind to DNA and make it easier or harder for RNA polymerase to bind to the DNA promoter.
Far-away clusters of binding sites for transcriptional activators and repressors, called enhancers and silencers, activate or repress genes in a certain cell or body part. This allows cells to be specialized for certain organ systems and parts of the body.
Why is gene expression important?
Gene expression and regulation are fundamental processes in the development, maintenance, and functioning of organisms, which are important to their overall health. They allow cells to adapt to different conditions, including environmental changes, stress, and disease. Proper regulation enables cells to respond appropriately to these different conditions. For example, when the body is exposed to a pathogen, certain genes associated with the immune response are activated to help fight the infection.
Gene expression regulation is also essential for maintaining the internal balance of the body, known as homeostasis. Genes involved in metabolism, hormone regulation, and energy balance are tightly regulated to ensure the body functions optimally.
Dysregulation of gene expression can lead to various diseases. Diseases can occur when mutated genes are expressed or when important genes are turned off. For example, uncontrolled cell growth due to changes in the regulation of genes involved in cell cycle control can result in cancer. Understanding and manipulating gene expression is crucial for developing therapies to treat or prevent such diseases.
Studying gene expression
Scientists can measure gene expression in a number of ways. Classically, scientists looked at gene expression by measuring protein activity. If protein activity is detected, the gene that encodes that protein is likely expressed. When, where, and how much a gene is expressed can also be assessed by measuring the functional activity of a gene product by observing a trait associated with a gene.
With advanced technology, scientists can now measure mRNA expression of every gene in the entire genome. Sometimes, they do that in individual cells. This is a really powerful tool to help measure which genes are turned on, how much, and where.
At the HudsonAlpha Institute for Biotechnology, gene expression and transcription factor biology have been at the center of much work since it opened. A group of scientists, led by Faculty Investigator Rick Myers, PhD, identified and studied hundreds of transcription factors through the Encyclopedia of DNA Elements (ENCODE) Project. In their most recent project, the team analyzed 680 transcription factors in a common cell line. Their work builds an important catalog of information about transcription factors, many of which haven’t been studied in depth before.
Faculty Investigator Nick Cochran, PhD, and his lab also study gene expression specifically in the context of brain health and disease. Many neurodegenerative diseases are characterized by dysregulation of gene expression that leads to toxic accumulation of proteins like tau and amyloid-beta in brain cells. The Cochran lab studies transcription factors and their binding sites in and near genes responsible for Alzheimer’s disease and other dementias. In a recent study, they took an in-depth look at regions of DNA that regulate the expression of the gene MAPT, which codes for the protein tau. Abnormalities in tau are associated with some of the symptoms of Alzheimer’s disease. The team, led by Bri Rogers, PhD, identified dozens of new MAPT enhancers that could help understand the disease more fully. The goal after identifying transcription factors bound at these enhancers is to use a variety of approaches to turn down the expression of mutated genes in hopes of mitigating the effects of the disease. Developing drugs to stop the transcription factors’ ability to turn on genes that drive Alzheimer’s disease pathology could prevent the onset or slow the progression of the disease.